The future of chemistry is at Carnegie Mellon
The four young faculty featured here are leveraging futuristic techniques and equipment to solve pressing problems affecting everyday life. Their research stands to have a significant impact in a wide range of fields, including health, biotechnology, energy storage, the environment and agriculture.
‘Tweezing’ with Light to Study the Chemistry of the Atmosphere
To better understand the pollution that travels through the vast expanse of Earth’s atmosphere and how it could affect our future, Associate Professor of Chemistry Ryan Sullivan is studying tiny particles just microns in size that float through the air we breathe.
What makes these particles so challenging but crucial to study is the fact that they evolve constantly in the atmosphere based on changes in the pollutant gases or other particles they encounter. This chemical evolution could change the particle’s future reactivity, or even amplify its harmful effects on human health or climate change.
For Sullivan, monitoring these particles in real-time means harnessing the power of lasers to trap and manipulate them. Laser beam, tightly focused with a microscope lens, holds the particles like a pair of tweezers, while also inducing a Raman vibrational spectrum from the particle that can be used to track its size, structure and chemical composition in real-time with high resolution.
Sullivan’s team is able to expose these tweezed particles to a variety of different substances to see what particles, called secondary organic aerosol particles, are formed in the reactions. He is the first scientist in North America to use optical tweezing, the creator of which was awarded the 2018 Nobel Prize in Physics, to study such organic aerosol particles.
“The optical tweezers really do allow us to directly probe or simulate how complex particles might actually evolve in the atmosphere,” Sullivan said. “We really don’t have other good ways of interrogating individual particles over hours of a reaction.”
Sullivan is continuing to pioneer more advanced ways of measuring particles, such as measuring how their pH evolves over time and how that alters their morphology and reactivity, and acquiring a mass spectrum to learn even more about their chemical composition.
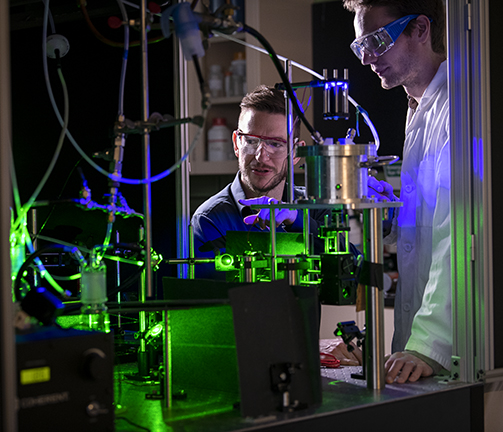
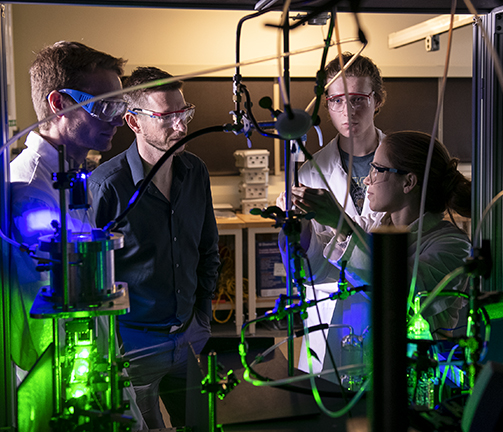
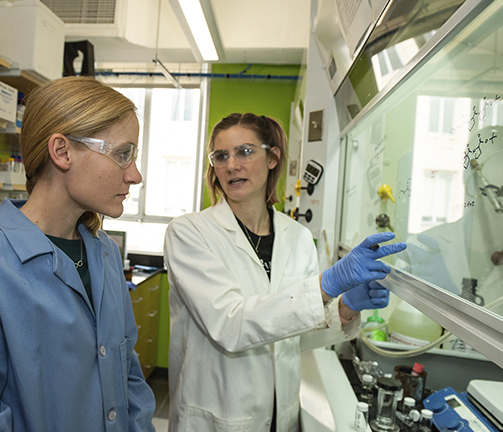
Healing Bones with Chemistry
After injuring the cartilage in her shoulder in graduate school, Assistant Professor of Chemistry Stefanie Sydlik got interested in the biological process of healing in our bodies. Now, her lab is pioneering the future of healing bones.
“Bone is a super interesting material in the body because we have the ability to regenerate it,” Sydlik said. “The question is: How do we tap into that natural healing response so we can heal major fractures the same as minor fractures?”
After suffering a severe bone fracture, many people require metal implants such as titanium plates to align their fractures properly and give them adequate blood supply to heal. However, these permanent implants can sometimes lead to long-term damage in the body, and they can’t be used in children who are still growing.
Sydlik’s lab has turned to graphite, the substance commonly used in pencils, as a better replacement. By modifying it with calcium phosphate, her team was able to create a material that avoids rejection by the body by mimicking the composition of natural bone and that degrades when it’s no longer needed. This material, which has been tested in mice, also releases chemical signals that instruct the body’s stem cells to heal a person’s bone in major fractures the same way they do for minor fractures.
Sydlik is now collaborating with researchers in Carnegie Mellon’s College of Engineering to 3D print this material into scaffolds with controllable geometry that could be implanted into any size or shape fracture. She envisions these scaffolds helping everyone from soldiers with devastating battlefield injuries to avid cyclists like herself.
“We’re using the power of chemistry to tell the biology what to do,” Sydlik said.
Powering the Future with Polymers
Hydrogen-powered cars, wind or solar power grids and your own phone or laptop — one thing all of these technological innovations have in common is the need to store and conduct large amounts of energy safely and efficiently.
“Energy storage is one of the biggest problems we have right now,” Associate Professor of Chemistry Kevin Noonan said. His lab’s research aims to help make energy storage and conduction devices that are cheaper, lighter and more efficient for a renewable future.
For example, the acidic conditions inside fuel cells usually require the use of metals like platinum as catalysts for the reactions that generate energy. However, these metals are heavy and expensive, making them not ideal for widespread implementation or for certain uses such as in vehicles. “It’s hard to run a car when you have platinum-based fuel cells,” Noonan notes.
“We’re really interested in whether we can make materials that conduct hydroxide anions so we can run fuel cells under basic conditions” Noonan said. These lightweight polymeric materials would enable the use of more abundant and cheaper metals like nickel to run these electrochemical cells efficiently.
Another area of his lab’s research looks at synthesizing new classes of conductive polymers derived from sugars. “We’re making electronic materials from bio-based resources,” Noonan said. These organic polymers could fill the role of the conductive metals currently used in everyday devices like LEDs and sensors, and even be used in interesting new ways like printed circuits or ultra-thin electronic displays. Crucially for Noonan, these polymers would be more sustainable than many environmentally unfriendly materials used now.
“I try to think about everything we do in a recyclable way. As we make new materials we have to think about sustainability and environmental impact.”
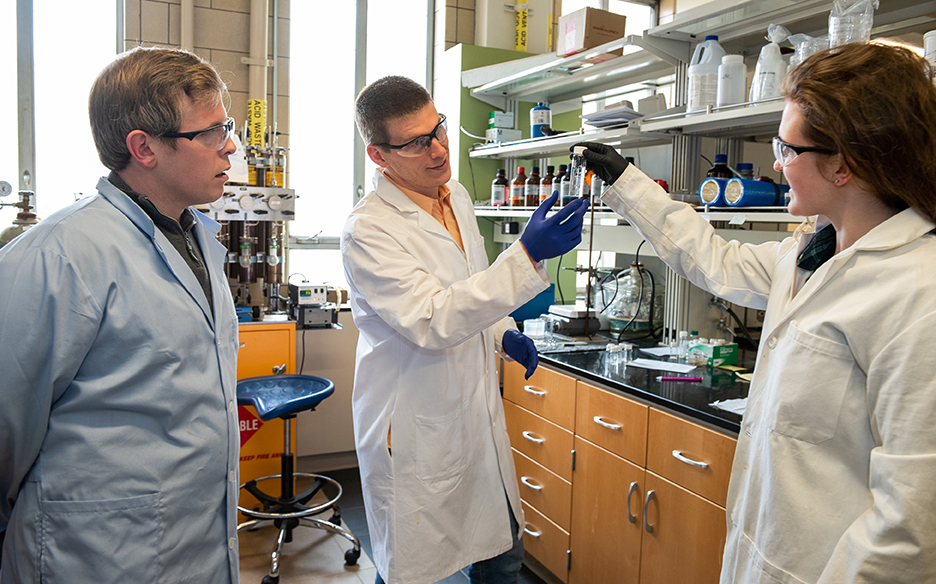
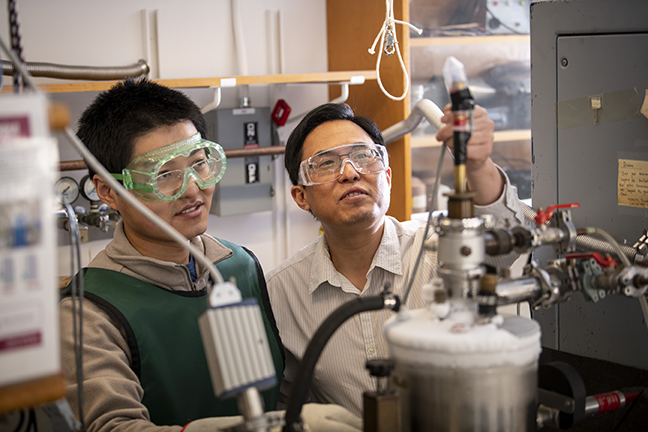
Catalyzing the Future
Many of the basic biological processes that fuel life on Earth involve intricate chains of chemical reactions with complex intermediates. While it’s usually easy to study the beginning and end of a reaction, the intermediate steps that enzymes take while performing these processes are often difficult to scrutinize. Assistant Professor of Chemistry Yisong (Alex) Guo is shedding light on these intermediate products to help scientists better understand how they work and how we can improve and take advantage of them.
Guo’s lab is particularly focused on enzymes containing metal ions, which often serve as key catalytic centers for reactions. Enzymes such as nitrogenases, oxygenases and hydrogenases are crucial for enabling reactions to break the very strong bonds of ambient molecular nitrogen (N2), oxygen (O2) and hydrogen (H2) and allow those molecules to be exploited. Using various advanced forms of spectroscopy, Guo is able to precisely map out the structures of various intermediate products in these chemical reactions.
Through better understanding of these natural catalysts and how they allow reactions requiring high amounts of energy to take place, Guo hopes that cheaper and more efficient synthetic catalysts could be developed based on chemical principles revealed in his research. These catalysts could be used to develop alternative fuels, pharmaceuticals or even fertilizers.
“For the growth of population in the future, you need more secured food supply, which means more fertilizers,” Guo notes. Many fertilizers are made from nitrogen, which is abundant in Earth’s atmosphere but locked in extremely strong bonds. It currently takes a metal catalyst and a lot of energy to break those bonds in the process of “nitrogen reduction” to make fertilizer. Deeper knowledge of how those natural metal-containing nitrogenase catalysts work may be the key to making catalysts that work better.
“Hopefully this knowledge can help people produce synthetic catalysts that can do nitrogen reduction much, much more efficiently,” Guo said.
♦ Ben Panko
Chemistry Faculty focus areas
Biomedical and Health Science Research
Bruce Armitage
Mark Bier
Marcel Bruchez
Subha Das
Roberto Gil
Yisong Guo
Michael Hendrich
Maria Kurnikova
Danith Ly
Krzysztof Matyjaszewski
Linda Peteanu
Stefanie Sydlik
Materials Discovery
Stefan Bernhard
Terrence Collins
Subha Das
Rongchao Jin
Hyung Kim
Tomasz Kowaleski
Krzysztof Matyjaszewski
Kevin Noonan
Linda Peteanu
Stefanie Sydlik
Newell Washburn
David Yaron
Earth and Sustainability Science
Stefan Bernhard
Terrence Collins
Neil Donahue
Yisong Guo
Rongchao Jin
Hyung Kim
Krzysztof Matyjaszewski
Kevin Noonan
Ryan Sullivan
Newell Washburn