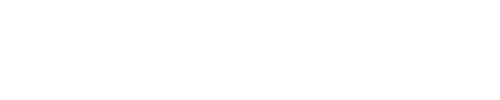
Physicists Help Solve ‘Scientific Mystery’ about Beta Decay
Since her time as a postdoctoral researcher, Assistant Professor of Physics Diana Parno has been involved with KATRIN, the international collaboration seeking to measure the mass of the neutrino.
To accomplish this, the KATRIN experiment takes precise measurements of the energy distribution of electrons emitted during the radioactive decay of tritium. However, KATRIN’s tritium is bound in T2 molecules — and the radioactive decay of tritium can excite not only the electron energy states of the molecule but also the vibrations and rotations of the molecule’s two nuclei.
“All of these excitations distort the spectrum that we measure, and we need a precise understanding of the excitations in order to correctly analyze the data,” Parno said.
KATRIN makes use of a theoretical framework to predict these excitations as well as how likely it is for the tritium molecules to dissociate following their radioactive decay. However, that framework’s predictions for dissociation contrast sharply with experimental measurements made in the 1950s.
In collaboration with colleagues at the University of Washington, Parno built the Tritium Recoil Ion Mass Spectrometer to precisely observe the beta decay of tritium gas and take a modern measurement. The results of those observations were published in the journal Physical Review Letters.
“Our results agree exactly with the theoretical prediction and indicate a design flaw in the 1950s experiments,” Parno concluded.
High-redshift quasar host galaxy & companion. Image: This artist’s illustration portrays two galaxies that existed in the first billion years of the universe. The larger galaxy at left hosts a brilliant quasar at its center, whose glow is powered by hot matter surrounding a supermassive black hole. Scientists calculate that the resolution and infrared sensitivity of NASA’s upcoming James Webb Space Telescope will allow it to detect a dusty host galaxy like this despite the quasar’s searchlight beam. Credit: J. Olmsted (STScI)
Simulation Proves Power of Webb Telescope
BlueTides, a powerful computer simulation developed by Professors Tiziana Di Matteo and Rupert Croft and run by physics graduate student Yueying Ni, has shown that NASA’s James Webb Space Telescope will be able to reveal the host galaxies of some distant quasars despite their small sizes and obscuring dust.
Quasars found at the centers of galaxies contain answers to many questions, including those about how black holes grow. For distant galaxies this can be hard to determine because their light has been stretched to longer wavelengths due to the expansion of the universe. As a result, light from distant galaxies’ black hole accretion disks or young stars get shifted to infrared wavelengths.
The Webb Telescope is equipped with infrared detectors that will enable it to see galaxies that are hidden from other telescopes. BlueTides was able to simulate the galaxies and quasars in the first billion years of the universe’s history. The simulations agreed with current observations and can be used to predict what Webb might see when it launches in 2021.
A diagram of the layer circular photogalvanic effect in quasi-two-dimensional chiral bilayers.
New Research Shows Promise of Tunable Two-Dimensional Materials for Light Detection
New research from Carnegie Mellon University shows how two-dimensional materials can be precisely tuned to act as sensitive detectors for a difficult-to-measure form of light.
“Material design sounds like a very complicated topic,” said Professor of Physics Di Xiao. “But deep down it’s just about how you arrange atoms.”
In a study published in the journal Physical Review Letters, Xiao and postdoctoral fellow Yang Gao showed how arranging two layers of graphene atoms can allow the detection of circularly polarized light.
Compared to the more familiar linearly polarized light, in which the waves of radiation move along a plane in a single direction, circularly polarized light occurs when the waves constantly rotate while moving along a plane in space. When polarized this way, light waves rotate left or right, leading to “left-handed light” and “right-handed light.”
“To be able to extract this polarization would be very important for many optoelectronic applications,” Xiao said, noting that it’s currently difficult and expensive to detect circularly polarized light, particularly in the infrared range.
A schematic of KRAS at the membrane at a most frequently assumed distance.
New Studies Highlight Innovative Contributions to Cancer Research
In two new collaborative studies, researchers in the Department of Physics’ biological physics group used advanced neutron scattering techniques to further the understanding of proteins crucial to the development of cancer.
“We have developed the scattering methodology, the preparation of suitable membrane mimics, the protein handling and the analysis of the scattering data to a point that we are now able to provide useful information to biomedical groups that work on important disease-related systems,” said Associate Research Professor of Physics Frank Heinrich of the work Carnegie Mellon University’s Supramolecular Structures Lab completed in collaboration with the National Institute of Standards and Technology.
Led by Professor of Physics Mathias Lösche, the lab contributed to two new studies seeking to better understand the biophysical foundation of cancer.
In research published in the Proceedings of the National Academy of Sciences, Heinrich and his collaborators were able to characterize the structure and dynamics of the signaling protein KRAS, a member of the Ras family of Small GTPase proteins located at the cell membrane that can mutate to contribute to the development of many cancers.
In another study published in Science Advances, the researchers characterized a different protein, ASAP1, which is essential for the activation of a distinct Small GTPase, Arf, a signaling protein that is also associated with a variety of human cancers.
Diagrams showing a topological superconductor created with layers of tungsten ditelluride and niobium diselenide.
Superconducting Material Holds Promise for Quantum Computing Student Stories
New research from Carnegie Mellon physicists details the creation of a special kind of superconducting material that could allow for the creation of new and more robust quantum computers.
“The main result is that we created a new state of matter,” said Assistant Professor of Physics Ben Hunt, who led the research in collaboration with Professor of Physics Randall Feenstra, Ph.D. candidate Dacen Waters and Felix Lüpke, currently a postdoctoral researcher at the Oak Ridge National Laboratory.
This state of matter, a one-dimensional topological superconductor, has actually been made before, Hunt clarified, but their study published in the journal Nature Physics proved its first creation in a particular material — tungsten ditelluride.
On its own, tungsten ditelluride is a fascinating material. When electricity is conducted through a sheet of it, instead of flowing evenly through the material, the electricity instead only flows around its edges. This unique conduction, described as one-dimensional, makes tungsten ditelluride a topological insulator.
“We knew that this state existed and what we did in this paper is that we took this really interesting edge state and made it go superconducting,” Hunt explained, by sticking the tungsten ditelluride onto the superconductor niobium diselenide.
Suter Leads Development of New 3D X-ray Diffraction Instrument
A consortium of scientists under the leadership of Professor Emeritus Robert Suter, in association with the Advanced Photon Source at the Department of Energy’s Argonne National Laboratory, have begun work on the next era of materials science research: the High-Throughput High-Energy Diffraction Microscopy Instrument (HT-HEDM).
The existing instrument, at beamline 1-ID, uses X-ray diffraction to provide measurements that give researchers a map of a material’s internal structure without destroying the material sample. This three-dimensional “X-ray vision” helps to refine models or theories of how materials behave when external conditions such as temperature or pressure are applied. Demand for such knowledge, which yields both fundamental new understanding and will aid in accelerating development of new applied materials, has expanded to exceed current measurement capacity.
The HT-HEDM instrument will be more efficient than its predecessor, while also being capable of taking more sensitive measurements and producing more streamlined analysis.
“We have updated various aspects of the original instrument, so this one will be faster and easier to run. Data reduction software will process multi-terabyte data sets and produce output data in as automated a way as we can manage,” said Suter. The consortium plans to have the device fully operational by January 2021.